Quantum Effects Of Photosynthesis Could Improve Energy Efficiency
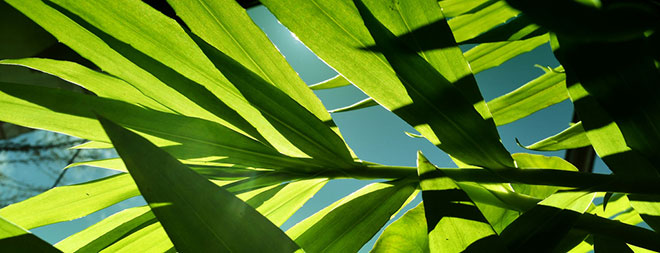
Joe Fanai via flickr | http://bit.ly/1dtDGCq
(ISNS) -- Sunlight drives nearly all life on Earth, and scientists want to develop ways for it to power civilization as well. Now researchers suggest that a relatively simple, biologically inspired technique for harvesting sunlight could in principle convert the sun's rays to electricity very efficiently.
In solar cells, molecules absorb photons, or packets of light energy, and give off electrons to generate an electrical current. However, these electrons can quickly combine with other charged particles, and get absorbed, causing the cells to lose efficiency. As the electrons are absorbed, the amount of electricity flowing through the cell is reduced.
On the other hand, the light-harvesting molecules that plants, bacteria and algae use in photosynthesis can convert light to electrical energy with nearly perfect efficiency under some conditions. This remarkable performance is impossible in classical physics — instead, experiments suggest it may be due to strange effects often seen in quantum physics. For instance, in quantum physics, particles such as electrons can essentially each be in more than one place at the same time or spin in two opposite directions simultaneously, a bizarre phenomenon known as superposition.
"It was very surprising to discover that biological systems like plants are actually using quantum mechanics to do things like photosynthesis," said researcher Andy Parker, a physicist at the University of Cambridge in England.
Scientists worldwide are investigating how photosynthesis works on a quantum level to design better solar cells. Now Parker and his colleagues have devised a relatively simple way for quantum effects to potentially significantly enhance artificial light-harvesting devices.
"We'd like to come up with a system that really can be built," Parker said. "We want to help address the energy crisis."
The researchers modeled a system made up of three molecules exposed to light, mimicking the architecture and molecular components seen in the photosynthetic pigments of plants. This scenario consists of two "donor" molecules that emit electrons after they absorb photons, and an "acceptor" molecule that receives the electrons given off by the donor molecules.
The scientists reasoned the donor molecules can interact with each other through their electromagnetic fields. "Atoms in the donor molecules have electrons around them, and those electrons can set up electromagnetic fields that the molecules can 'see' across the distance between them," Parker said. "It's a lot like how two magnets can 'see' each other across distances — if one is aligned one way, the other will tend to align the same way."
This interaction between the donor molecules leads them to share electrons. A strange principle of quantum physics then comes into play known as quantum interference, where particles such as electrons can behave like the waves seen rippling on the surfaces of ponds, interfering with each other in complex ways.
Quantum interference leads the donor molecules to both become good at absorbing light and bad at recombining with electrons they give off. Calculations suggest this system could generate 35 percent more current than a solar cell that works based on classical physics alone.
This model the researchers propose is simpler than some more exotic ones proposed for how photosynthesis works. "We're saying relatively straightforward effects can produce real benefits," Parker said.
"This is only a theoretical paper with a 'toy model,' yet it incorporates some nice ideas that may be advantageous for future molecular designs," said chemical physicist Elad Harel at Northwestern University in Evanston, Ill., who did not take part in this research.
Parker cautioned they are not saying this system is definitely how plants achieve such amazing efficiency with photosynthesis, but one element of what plants might do.
"The molecules involved in photosynthesis are really quite complicated, and we don't want to extrapolate from a few simple mechanisms to saying we completely understand a highly evolved system such as photosynthesis," Parker said.
The researchers add they have not actually built this system in real life yet. "However, we know there are molecules with these general properties that can be made in the lab, and we're talking with people who work in this area to come up with a system," Parker said.
Harel cautioned "the biggest problem is that these model systems are extremely difficult to synthesize." There may be many ways in which energy can get lost "that prevent the type of efficiencies reached by these schemes," Harel added. "In other words, real systems are much more complex."
More realistic simulations of actual systems are critical, Harel said. "What specific molecules will be used? What is their structure? How do they fluctuate and move at room temperature? Are they in solution or in a film?" Harel asked.
Parker and his colleagues detailed their findings Dec. 18 in the journal Physical Review Letters.