Update on Trapping Antimatter Atoms
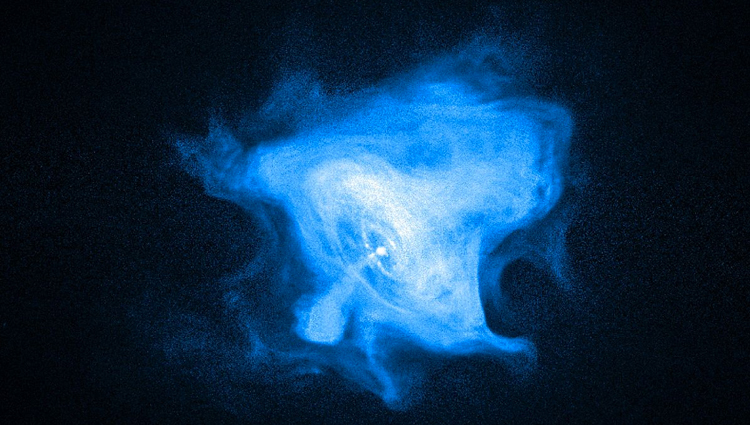
Antimatter is the stuff of science fiction, but it is also occupies the center of many of the most pressing unsolved physics questions of our day. This afternoon, I attended a seminar by Harvard University's Phil Richerme at the Joint Quantum Institute on the University of Maryland campus providing an update on one of the difficult modern-day physics accomplishments: making antimatter versions of entire atoms. Anti-atoms have been made for about a decade now, but scientists are trying to move to the next step: holding and studying them for long periods of time, an endeavor that promises many new insights in physics.
In 1928, physicist Paul Dirac discovered, in the act of writing equations, that the electron, the basic negatively charged particle known for carrying electricity, had an antimatter counterpart, seemingly identical except for the fact that it had an opposite charge. The electron's antimatter cousin is called the positron. If an electron came into contact with a positron, they would destroy each other and create a burst of energy.
Dirac, who won the Nobel Prize in 1933 for his discovery, also realized that all known particles have antimatter counterparts. Protons, the positively charged particles that occupy the core of atoms, have antiprotons. All these antiparticles were discovered and studied for decades. Scientists discovered that antimatter can occur naturally--for example, cosmic rays from space contain positrons that travel to the Earth. Antimatter can also be created in the laboratory.
A fundamental question of physics today is: Why is there so much more matter in the universe than antimatter? When our universe began, basic physics principles suggest that equal amounts of matter and antimatter should have been created. This matter and antimatter should have annihilated each other, leaving no matter or antimatter. But there was a slight imbalance of matter over antimatter, and therefore, there is matter in the universe, allowing us to exist.
To understand this imbalance, physicists are trying to see if there are tiny differences between matter and their antimatter counterparts. Antimatter particles have been studied for decades, but entire atoms of antimatter--an antimatter electron and an antimatter proton--had not been made. It's one of the most difficult things to do in all of science.
About a decade ago, scientists at the CERN accelerator in Europe succeeded in making antihydrogen atoms, atoms made of a positron and an antiproton. Researchers slam protons into a metal target, and the collision creates fast, high-energy antiprotons. Then they direct these antiprotons to a trap that confines them with electric and magnetic fields. They slow down the antiprotons so they become "cold" and try to combine them with positrons. Every influx of antiprotons, and attempt to pair them with positrons, is called a "trial." Creating these anti-atoms is extremely challenging in part because the anti-protons have to slow down greatly, to near absolute zero, and engage in a gentle dance with the positrons in order to get them to join in a successful union as anti-atoms. And once they become anti-atoms, their net electric charge is zero, making them harder to keep in a trap and hold them for observation.
In 2002, both the ATRAP collaboration, of which Richerme is part, and the ATHENA collaboration at CERN produced and observed small numbers of antihydrogen atoms. But for physicists, creating them was only the first step. They wanted to be able to trap and study sufficient numbers of antihydrogen atoms for sufficiently long times so they can understand if they have any differences from hydrogen atoms. Hydrogen is the simplest atom, with only one electron and one proton, and antihydrogen is the simplest anti-atom, with a positron and antiproton. Researchers would like to determine if antihydrogen and hydrogen have the exact same internal structure, namely their energy levels, which should be the same. Does gravity affect hydrogen and antihydrogen differently? If there are differences, this could point to possible ways in which antimatter had a less fortunate fate in our early universe.
Fast forward 10 years, and "cold antihydrogen production is now a routine thing," Richerme said.
In the past year, a collaboration called ALPHA produced approximately 300 anti-atoms in about 300 trials and held them for up to 1000 seconds. ATRAP also reported that it trapped approximately 100 anti-atoms in 20 trials and kept them for up to 1000 seconds. Richerne said that antiprotons at CERN are being cooled to new low temperatures of 3.5 Kelvins, or 3.5 degrees above absolute zero, making them easier to pair with positrons to form anti-atoms. Richerme said that if they are able to hold 1000 atoms for the times they are trapping them now, they could perform measurements of certain properties such as energy levels in the antihydrogen to a precision of one part in a trillion, so that they can begin to test certain theories about matter and antimatter in the universe. Towards these ends, Richerme said ATRAP is developing a new, more sensitive antiatom-trapping apparatus, which they plan to commission this year, that should be better able to hold and observe antihydrogen atoms.
Physicists win either way. If they find no differences between hydrogen and antihydrogen properties, they can "constrain" or limit differences between the two, and they can accordingly tighten their theories and look for different explanations of the matter-antimatter balance beyond differences in the particles themselves. If they find differences, this could point the way to new physics phenomena that may be responsible for differences in them.
In either case, cold antihydrogen is one of the hottest things in physics to watch.